Optimizing Plate Heat Exchanger Transfer Efficiency (Part Two)
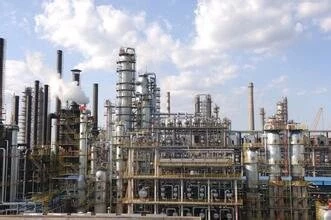
1. Improving Heat Transfer: Finding the Right Balance
Enhancing the average flow rate within the flow channel has the potential to augment the heat transfer coefficient and diminish the heat exchanger area. Nevertheless, this comes with trade-offs such as increased heat exchanger resistance, elevated power consumption by the circulating pump, and heightened equipment costs. Given that the power consumption of the circulation pump is proportional to the third power of the medium flow rate, pursuing a slightly higher heat transfer coefficient through increased flow rate may prove uneconomical.
2. Smart Moves for High Flow: Hot Mixing Plates
In scenarios where the flow rate of both hot and cold media is substantial, strategies can be employed to mitigate heat exchanger resistance and ensure a high heat transfer coefficient. One approach involves the use of hot mixing plates, characterized by symmetric geometric structures on both sides. These plates can be categorized based on the angle of herringbone-shaped corrugations, offering different heat transfer coefficients and fluid resistances. The combination of plates with varying angles can create three distinct flow paths (HH, HL, LL) tailored to diverse working conditions.
3. Choosing Designs: Symmetry vs. Asymmetry
However, caution is advised when the flow ratio of hot and cold media is excessively large, as the pressure loss on the side of the cold medium can be significant, and precise plate matching may pose challenges. In such cases, employing hot mixing plates is not advisable. Another option is the symmetrical plate heat exchanger, featuring plates with identical corrugated geometry on both sides and equal cross-sectional areas for hot and cold flow channels.
For scenarios where the flow rate of hot and cold media is considerable, the asymmetric plate heat exchanger, with different flow cross-sectional areas, offers a viable solution. While the heat transfer coefficient may slightly decrease, the pressure drop is significantly reduced. In such asymmetrical configurations, a single flow can reduce plate area by 15% to 30% compared to symmetric single flow heat exchangers.
4. Balancing Flow Channels: Multi-Flow Strategies
Alternatively, in instances of large flow rates, a multi-flow combination arrangement can be adopted. This involves using more channels on the side with a smaller flow to increase flow rate and achieve a higher heat transfer coefficient, while employing fewer channels on the side with larger flow to reduce heat exchanger resistance.
5. Tackling Large Flows: Bypass Pipes to the Rescue
To address challenges related to large flow rates, bypass pipes can be introduced at the inlet and outlet of the heat exchanger on the larger flow side. These pipes, equipped with regulating valves for convenient adjustment, help reduce flow rate and resistance. A counter-current arrangement in the bypass pipe ensures a higher temperature of the cold medium heat exchanger, meeting design requirements after flow merging on the outlet. While effective in enhancing heat transfer coefficient and reducing resistance, this method may involve slightly complicated adjustments.
6. Ideal Conditions: Flow Speed and Resistance
It is recommended to maintain an average flow velocity between 0.3-0.6 m/s and a resistance not exceeding 100 kPa within the flow path. The selection of plate heat exchangers, whether symmetrical or asymmetrical, single-flow or multi-flow, along with the use of bypass pipes, should be based on detailed thermal calculations considering the flow ratio of different cold and heat media.