Material Selections for Cryogenic Pressure Vessel Design (Part Two)
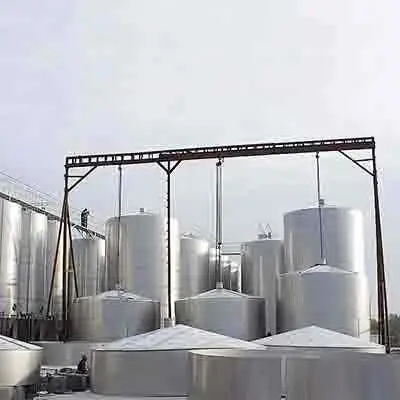
2. Chemical Composition
In the realm of cryogenic pressure vessels, adjusting the carbon content plays a crucial role in influencing material brittleness and the brittle transition temperature of the pressure vessel. To enhance low-temperature steel resilience, it is recommended to keep the carbon content below 0.2%. A contemporary trend favors the utilization of low carbon (< 0.15%) or micro carbon (< 0.06%) steel for improved performance.
Manganese acts as a pivotal element, expanding the austenite region and contributing to the development of fine and robust ferrite and pearlite grains. This, in turn, enhances steel toughness at low temperatures. Manipulating the manganese-iron ratio impacts the nil-ductile transition temperature, providing flexibility in optimizing the steel's performance at varying carbon content levels.
Additionally, nickel proves superior to manganese in improving low-temperature toughness, with 3.5% nickel content maintaining high toughness at -100 °C, and 9% nickel rendering steel resistant to temperatures as low as -196 °C, suitable for liquid nitrogen containers.
3. Grain Size of Steel
The grain size of steel significantly influences its susceptibility to low-stress brittle fractures. A finer grain not only increases fracture strength but also lowers the brittle transition temperature. Fine grain structures exhibit reduced brittle phase per unit area, elevated surface energy, and diminished probability of crack occurrence, thereby improving low-temperature resistance. Furthermore, fine grain steel demonstrates uniform performance, lowering the brittle transition temperature.
4. Inclusions in Cryogenic Steel
Phosphorus and oxygen in steel contribute to grain boundary segregation and oxide precipitation, elevating the brittle transition temperature and fostering low-stress brittle fractures. Effective deoxidation, such as in killed steel, enhances low-temperature toughness. Silicon, aluminum, or aluminum and titanium (vanadium, niobium) further refine the grain and improve low-temperature toughness. Sufficient deoxidation reduces gas content, spheroidizes inclusions, minimizes dislocation accumulation, and lowers the brittle transition temperature.
5. Heat Treatment
Heat treatment significantly impacts the susceptibility of steel to low-stress brittle fractures. Quenching and tempering methods enhance ferrite and granular carbide structures, improving low-temperature toughness. However, precise control of tempering temperatures is crucial to prevent adverse effects on toughness. Normalizing is a common heat treatment for cryogenic steel, with adjustments based on alloying elements. Annealing is avoided in cryogenic pressure vessels, and post-weld heat treatment should adhere to specific temperature limits. Heat treatment inhibits brittle phase precipitation, alters precipitated phase characteristics, enhances steel strength, and effectively prevents crack expansion through the retention of austenite or ferrite in the tempered structure (tempered martensite).
Optimizing cryogenic steel involves strategic adjustments in chemical composition, grain size, inclusions, and heat treatment. Careful control of carbon content, utilization of elements like manganese and nickel, grain refinement, and effective deoxidation contribute to improved low-temperature toughness. Precise heat treatment, including quenching and tempering, ensures resilience against low-stress brittle fractures. This comprehensive approach ensures cryogenic pressure vessels maintain structural integrity and reliability in demanding low-temperature conditions.